Earthquake Management in Iran A compilation of literature on earthquake Management Iranian Studies Group at MIT Draft: January 6, 2004
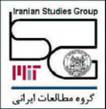 Introduction Less than ten days ago, a deadly earthquake devastated the Iranian city of Bam, killing more than 30,000 people and injuring another 50,000. It was one of the deadliest natural disasters in modern Iranian history, and similar in destruction to the Roudbar earthquake in northern Iran in 1990. Historical information and all available records show that approximately 130 large earthquakes have taken place in most parts of Iran. Considering the high seismicity of Iran, a comprehensive hazard reduction program was launched in Iran in 1991, but the effectiveness of the measures have been limited by lack of adequate funding and institutional coordination. There is no lack of probabilistic studies on the seismicity of the country as well as fault studies. Comprehensive studies have been performed by Berberian (1999, 2001), Amiri et al. (2003), Sarkar et al. (2003), Modarressi (2002), Walker (2002) and Tehranizadeh (2001, 2002). A more detailed bibliography is presented at the end of this document. A closer analysis of what transforms a natural event into a human and economic disaster reveals that the fundamental problems of development that the region faces are the very same problems that contribute to its vulnerability to the catastrophic effects of natural hazards. The principal causes of vulnerability in the region include rapid and uncontrolled urbanization, the persistence of widespread urban and rural poverty, the degradation of the region's environment resulting from the mismanagement of natural resources, inefficient public policies, and lagging and misguided investments in infrastructure. Development and disaster-related policies have largely focused on emergency response, leaving a serious underinvestment in natural hazard prevention and mitigation. A proactive stance to reduce the toll of disasters in the region requires a more comprehensive approach that encompasses both pre-disaster risk reduction and post-disaster recovery. It is framed by new policies and institutional arrangements that support effective action. Such an approach involves the following set of activities: • risk analysis to identify the kinds of risks faced by people and development investments as well as their magnitude; • prevention and mitigation to address the structural sources of vulnerability; • risk transfer to spread financial risks over time and among different actors; • emergency preparedness and response to enhance a country's readiness to cope quickly and effectively with an emergency; and • post-disaster rehabilitation and reconstruction to support effective recovery and to safeguard against future disasters. Though it is not possible to completely avoid the natural disasters, the sufferings can be minimized by creating proper awareness of the likely disasters and its impact by developing a suitable warning system, disaster preparedness and management of disasters through application of information technology tools. This becomes particularly important for the Iranian society, as the prospect for a major earthquake in Tehran with potential casualty rates in the hundreds of thousands creates a sobering wake-up call for government officials to take a more proactive stance. The Iranian Studies Group January 2004 Earthquakes: The Most Destructive Force of Nature Earthquakes are the most destructive among all the natural hazards. Most of the time, they occur without any warning, which makes them most feared and unpredictable natural phenomena. Globally, on an average two earthquakes of magnitude 8 are known to occur every year. Iran is surrounded by tectonically active zones. Earthquakes are regularly felt on all sides of Iran. But the capital, Tehran, has been fortunate enough to avoid a major quake this century. Tehran was shaken in 1830 by a magnitude 7.2 quake. As can be observed from Figure 1, Iran is one of the most seismic countries in the world. About 15,000 people died in 1976 as a result of an earthquake in western Iran. In 1981, more than 1000 people dies in Kerman as a result of an earthquake. Around 40,000 people died in a 7.2 Richter scale earthquake in the northern Iranian province of Gilan. In June of 2002, more than 1100 people lost their lives in an earthquake in Northwestern Iran. The casualties of the Bam earthquake of December 2003 are estimated to be between 30-50 thousand. The earthquake in Bam is one of 130 major earthquakes that have happened in recorded history of Iran. A map of the earthquake area can be seen in Figure 2. 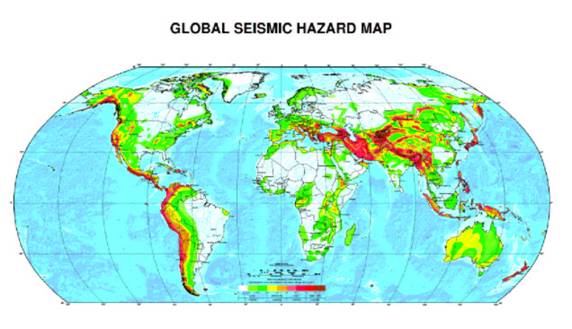 Figure 1. Global Seismic Hazard map Source: GLOBAL SEISMIC HAZARD ASSESSMENT PROGRAM, Switzerland
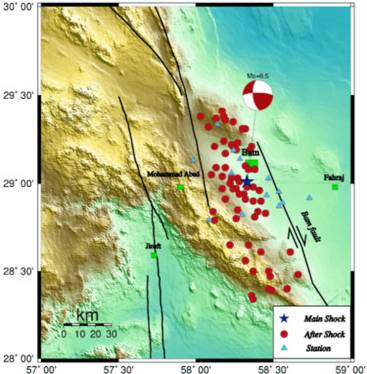 Figure 2. Bam Earthquake zone (Source: IIES) Earthquakes: Causes The true nature of the causes of an earthquake must be fairly well understood before adopting any control measure. Two models are being tested to justify these control measures. 1. Dilatancy-diffusion theory developed in the U.S. 2. Dilatancy-instability theory USSR The first stage of both models is an increase of elastic strain in a rock that causes them to undergo a dilatency state; which is an inelastic increase in volume that starts after the stress on a rock reaches one half its breaking strength. During Dilatancy State, open fracture developing the rocks. So it is in this state the first physical change takes place indicating future earthquake. Here the two models diverge. The U.S. model suggest that the dilatancy and fracture of the rocks are first associated with a low water containing dilated rock, which helps in producing lower seismic velocity, lower electrical resistivity and fewer minor seismic event. The pore water pressure then increases due to influx of water into the open fracture, weakening the rock and facilitating movement along the fracture, which is recorded as an earthquake. In contrast the Russian model state that the first phases is accompanied by an avalanches of fracture that release some stress but produce an unstable situation that eventually cause a large movement along a fracture. Seismic gaps are defined as an area along active fault zones, capable of producing large earthquake but that have not recently produced an earthquake. These areas are thought to store tectonic strain and thus are candidate for future large earthquake. Any fault that has moved during quaternary can be called as active fault. It is generally assumed that these faults can get displaced at any time. Faults that have been inactive for the last three million years are generally classified as inactive fault. Active faults are basically responsible for seismic shaking and surface rupture (Sinha et al. 2000) Like all other natural hazards earthquakes also produce primary and secondary effects. Primary effects include surface vibration, which may be associated with surface rupture and displacement along fault plane. These vibrations may sometimes lead to the total collapse of large buildings, dams, tunnels, pipelines and other rigid structures. Deterministic ground motion analysis is one of the tools to determine the spatial distribution of surface vibration. Secondary effects of earthquake include a variety of short-range events; such as liquefaction, landslides, fires, tsunamis and floods. Long-range effects include regional phenomena such as regional subsidence or emergence of landmasses, river shifting and regional changes in ground water level. Liquefaction results from transformation of water-saturated granular material from solid to liquid state as a result of increase in pore water pressure. It may result in three types of failure. Earthquakes: Primary and Secondary Effects a. Landslide on moderate slope. b. Landslide occurring on gentle or nearly flat slopes. c. Quick condition failure. Landslide of all varieties in addition to those associated with liquefaction, are triggered or directly caused by earthquake. Earthquakes also cause catastrophic destruction from fires due to disruption of electrical power lines and broken gas lines. Coastal or submarine earthquake also generate tsunamis or seismic sea waves. Other secondary effects of large earthquake are regional changes in land elevation. The destruction of critical facilities may cause catastrophic losses of life, property damage or disruption of society, e.g. large dams, nuclear power plant and liquid natural gas. Earthquakes: Economic Impacts As described by Munich Re,(1,2) in comparison to the decade of the 1960s, the frequency of severe natural catastrophes has increased by a factor of three and the direct economic costs have increased by a factor of nine. Although the direct damages of approximately $70 billion a year from natural disasters seem evenly split between the developed and developing (including transition) countries, the per capita impact on the developing or transition countries is nearly 20 times as great as in the developed world. Concern about these costs has generated considerable activity in the international development community. The United Nations declared the 1990s the International Decade of Natural Disaster Reduction. The World bank created the Disaster Management Facility to direct the bank's responses to disaster losses. Each of these organizations has spawned a series of activities focused on natural disasters and developing and transition countries. In most countries, reconstruction of damaged infrastructure is financed by postevent measures. These measures usually include increased borrowing or diversion of existing budgeted funds to finance reconstruction. The World Bank has provided $14 billion in post-natural-disaster reconstruction financing during the past 20 years.(3) This is nearly 250% of the amount provided for reconstruction work following civil disturbances. During the past decade, the scientific understanding of the causes and consequences of natural catastrophes has dramatically improved. Models to estimate the frequency and severity of catastrophic events have been coupled with techniques to measure the vulnerability of capital stock to catastrophe losses. This has not only improved the probability estimates of natural catastrophes, but also of the potential consequences of these events. Although sophisticated modeling of both the nature and costs of natural catastrophes exists for developed countries, little reliable data on the risks of natural catastrophes has been developed for developing and transition countries. Furthermore, in the developed world, the tool used for complex financial planning to absorb the costs of catastrophes is modeling. Today, insurance absorbs more than half of the economic losses from natural catastrophes in the developed world. Also, the use of catastrophe-insurance-related tools has been increasing significantly in recent years. In contrast, less than 2% of losses from natural disasters are insured in the developing world. Earthquakes: Improving unreinforced masonry with FRP Structural weakness or overloading, dynamic vibrations, settlements, and inplane and out-of-plane deformations can cause the failure of unreinforced masonry (URM) structures, such as those in the Bam area. In the case of overstressing, these buildings have features, such as unbraced parapets, inadequate connections to the roof, floor, and slabs, and the brittle nature of the URM elements that can threaten human lives. Organizations such as The Masonry Society (TMS) and the Federal Emergency Management Agency (FEMA) have determined that failures of URM walls result in more material damage and loss of human life during earthquakes than any other type of structural element. Post-earthquake observations in Northridge, Calif. (1994) and Izmit, Turkey (1999) proved this point. Externally bonded fiber reinforced polymer (FRP) laminates and bars have been successfully used to increase the flexural and/or the shear capacity of reinforced concrete for retrofitting of the civil infrastructure. FRP composites also can provide solutions for the strengthening of URM walls that are subjected to inplane and out-of-plane overstresses caused by high wind pressures or earthquake loads. Available test results show that each potential failure cause of URM walls is prevented and/or lessened by using FRP composites. The most important characteristics of a strengthening work are the predominance of labor and shutdown costs, as opposed to material costs, time, site constraints, and long-term durability. The advantages of FRP versus conventional steel for use as a reinforcement include higher strength, lower installation costs, improved corrosion resistance, on-site flexibility of use, and minimum changes in the member size after repair. FRP material systems are composed of fibers embedded in a polymeric matrix and exhibit several properties which make them suitable for use as structural reinforcing elements. FRP composites are characterized by excellent tensile strength in the direction of the fibers and by negligible strength in the direction transverse to the fibers. Composites do not exhibit yielding, but instead are elastic up to failure. They are also characterized by a range of low to high modulus of elasticity in tension and low compressive properties. FRP composites are corrosion resistant and perform better than other construction materials in terms of weathering behavior. The FRP matrix consists of a polymer (or resin) used as a binder for the reinforcing fibers. The matrix has two main functions: enable the load to be transferred among fibers and protect the fibers from environmental effects. In a composite material, fibers have the role of the load-bearing constituent. Fibers give the composite high tensile strength and rigidity along their longitudinal direction. Several types of fibers have been developed for use in FRP composites. For structural applications, research and development have been conducted using carbon, aramid, and glass fibers. In the order listed, these fibers exhibit an ultimate strain range of 1 to 4% with no yielding occurring prior to failure. The ultimate strength range is about 830 to 480 ksi and the elastic moduli range is from 39,000 ksi to 10,000 ksi. FRP composites are available in the form of pre-cured laminates or fiber sheets installed by hand lay-up. The application of the latter offers several advantages, such as ease of bonding to curved or irregular surfaces, lightweight, and the fibers can be oriented along any direction. As a point of reference, the thickness of an installed ply (which includes fibers and adhesive) is in the range of 0.04 to 0.12 inch. FRP composites are also found in bar form. In certain cases, FRP bars are more convenient than using FRP laminates because of anchoring or aesthetic requirements. The bars are manufactured using a pultrusion process. Typical fiber content is 75% by weight. Sizes between #2 and #10 are commercially available. Application of near-surface-mounted (NSM) FRP bars does not require any surface preparation work and requires minimal installation time, compared to FRP laminates. Another advantage is the feasibility of anchoring these bars into members adjacent to the one being strengthened. For instance, in the case of strengthening masonry infill with FRP bars, they may be easily anchored to columns and beams. The photo on page 32 illustrates a variety of carbon FRP (CFRP) and glass FRP (GFRP) bars having round and square sections. Strengthening techniques There are two FRP applications for strengthening URM walls using laminates and bars. The first application deals with the improvement of capacity to withstand out-of-plane loads (flexural strengthening). The second application is to improve the strength of in-plane loads (shear strengthening). FRP laminates are formed by manual lay-up onto the surface of the member being strengthened. Prior to the fiber ply installation, the masonry surface may need to be prepared by sandblasting, application of a primer, and puttying, depending on its conditions. The first coat of resin is then applied, followed by the fiber ply which is impregnated by a second coat of resin. After hardening, the newly formed laminate becomes an integral part of the strengthened member. In another form of hand lay-up, a pre-impregnated ply is applied directly to the masonry surface. Two layers of resin are applied. The second layer is brushed on after the fiber has been adhered to the wall. Earthquakes: Hazard Reduction through Early Detection For any modern nation public safety concern are of prime importance and Iran is of no exception. In view of this concern there should be adequate thrust towards understanding earthquakes the science of which is known as seismology. These studies may be helpful in seismic hazard assessment and mitigation A comprehensive earthquake hazard reduction programme should include a. Prediction b. Control measure. c. Post earthquake rehabilitation measure. Prediction: First successful prediction of a major earthquake was made in 1975. The earthquake took place in China ( Haichung) on Feb 4 1975. The intensity of the earthquake was 7.3 on richter scale and about ninety percent of the structure was destroyed in a city of 90,000 people. In this case thousand of people were saved by the massive evacuation from unsafe housing just before the earthquake. The short-term prediction was possible primarily on a series of foreshock that began four days prior to the main shock. Unfortunately these types of short-range prediction on the basis of foreshock are not always reliable. Earthquake prediction by any geoscientist is far from success; however a detailed and systematic investigation may lift haze in its prediction. Earthquake prediction in an area may be carried out under following heads: a. Lithological characterization and structural setting of the region b. Crustal deformational studies c. Frequency of fore shock d. Repetitive land level survey e. Water tube tiltmeters f. Geomagnetic observation. g. Geothermal gradient. h. Gravity survey i. Hazard mapping Lithological Characterization and Structural Setting of the region Geological mapping of an area is the first step towards the surface and subsurface investigation of a region. The accuracy of these investigations decides the prediction accuracy before an earthquake and also the post earthquake control and reduction measures. The advent of Geoinformatics has brought revolutionary change in these investigations. Now a day number of geological softwares are available in the market for the geological mapping of the area. These softwares are highly useful in the speedy and accurate execution of mapping work. The arrival of GPS has the capability of recording spatial co-ordinates with accuracy level up to millimeter. Remote sensing and air photogrammetry is of immense potential at the reconnaissance stage of the mapping. Now it is possible to map the inaccessible regions through the satellites. Structural setting indicates the future earthquake by giving enough information regarding the palaeoseismology of the area. It is also helpful in the hazard mapping of the area to take preventive and control measures. "Earthquake don't kill people, but the unsafe building". Carrying out systematic lithological mapping of the terrain can very well minimize the magnitude of seismic destruction. It has been observed that the structures on a consolidated foundation, e.g. igneous and metamorphic rocks, are safer than those on unconsolidated basements, viz. Alluvial, sand and loamy soil. Different surface materials behave differentially in response to seismic shaking of various frequencies. Unconsolidated earth materials (mud alluvium and bedrock) vibrate more in compare to hard bedrock. Therefore an area sensitive to earthquake hazards must be mapped for it to be available to land-use decision maker. So a highly accurate geological map can be prepared with the help of recent geomatic tools and they can be analysed through GIS to use it for the prediction or the preparation of action plan during the post earthquake rehabilitation measures By predicting the intensity of shaking due to an earthquake before the earthquake occurs we can help plan to prevent damage. Doing this rapidly after an earthquake can help manage the emergency response efforts. Intensity is a measure of the effects of earthquakes. Examples include damage to man-made structures, ground failure and felt shaking. Intensity is not the same as magnitude, although it is influenced by earthquake magnitude. Intensity is also influenced by distance from the fault, ground conditions, and, sometimes, directivity. The most commonly used intensity scale today is the Modified Mercalli Scale. This is the scale we have used in the predictive models. These predictive intensity maps have been generated following the method described in the 1995 ABAG report "On Shaky Ground" by Jeanne Perkins and John Boatwright Crustal Deformation Studies through GPS Throughout the world most of the earthquake activity are confined to plate margin associated with crustal deformation. Now a day’s crustal movement can be recorded with high degree precision using GPS. Crustal deformation through GPS is one of the fast emerging area and most probably the only area where the real potential of GPS lies as far as effective earthquake prediction are concerned. GPS is now being used effectively for monitoring of crustal movement. In modern time, earthquakes are studied with more authenticity, as high quality seismic and geodetic data are available globally. Progress in this field with the establishment of Broad band digital seismograph and geodetic network. Data accumulated through the seismological, geological and geodetic observations can be of great help in the delineation of the earthquakes prone areas. This will have direct impact on the hazard assessment and public safety measures. Frequency of Foreshock There are cases where minor foreshocks have indicated the major coming shock. So it is highly desirable to establish the seismological observatory for the continuous monitoring of seismic activity in an area. In modern time, earthquakes are studied with more authenticity, as high quality seismic and geodetic data are available globally. Data accumulated through the seismological, geological and geodetic observations can be of great help in the delineation of the earthquakes prone areas. This will have direct impact on the hazard assessment and public safety measures. On that basis countries can be divided into 5 zones with respect to intensity of earthquake. Of these, zone v is seismically the most active where earthquake of magnitude 8 or more could occur. Zone I is the least active region. There are various parameters, which can be analyzed collectively for the purpose of an earthquake prediction. Surface parameter include topographical changes and subsurface parameter include subsurface geomagnetic, geothermal and gravity variation. Rates of uplift and subsidence, specially rapid or anomalous change may be significant in predicting earthquake. For example for more than ten years before the 1964 earthquake near Nigata, Japan there was anomalous uplift of the earth crust. It has also been observed that speed of primary waves may decrease for a month, and then increase to normal just before an earthquake. With recent advancement in science & technology, a proper sensor developed to measure these variations can be put on satellite to get regional idea by periodic and continuous monitoring of these variations and their quantification may helps us in forecasting of such hazardous events. Changes in the electrical resistance of an area have also been reported before earthquake. Increase in the amount of radioactive radon gas that is dissolved in deep well water has also been reported. The main objective of earthquake preventive measures should be to develop and promote knowledge, practices and policies that reduce fatalities, injuries and other economic losses from earthquake. Providing Geoscientific information to the masses can well minimize these losses. Formulation of preventive measures includes: Earthquakes: Preventive Measures i. Compiling digital surfacial geological maps to find out area more prone to crustal movement. ii. Preparation of ground shaking amplification maps to demarcate area susceptible to high amount of destruction. iii. Preparation of liquefaction and lateral spreading susceptibility maps iv. Preparation of landslide susceptibility maps mainly in high relief region to delineate area highly susceptible to landslide v. Compilation of GIS databases of existing data on active earthquake source zones and make these databases easily accessible to user groups vi. Modification of palaeoseismological maps vii. Geoscientific modeling of the shallow crust using seismic, geodetic and geological data for earthquake hazard evaluation. Earthquake hazard prevention depends on proper understanding of the destructive effect of earthquake. The areas of interest in earthquake studies include the effect of the earthquake source, local and regional geological structure and near surface geological deposits on strong ground shaping. Seismic and crustal deformation monitoring network can provide real time information for emergency response. GIS database of active earthquake source zone with up to date information on slip-rate and recurrence intervals will improve earthquake hazard identification and risk assessment. The Use of Information Technology GIS provides a tool for effective and efficient storage and manipulation of remotely sensed data and other spatial and non-spatial data types for both scientific management and policy oriented information. This can be used to facilitate measurement, mapping, monitoring and modeling of variety of data types related to natural phenomenon. The specific GIS application in the field of Risk Assessment are:- Hazard Mapping to show earthquake, landslides, floods or fire hazards. These map could be created for cities, districts or even for the entire country and tropical cyclone Threat Maps are used by meteorological departments to improve the quality of the tropical storm warning services and quickly communicate the risk to the people likely to get affected by the cyclone. Remote Sensing is considered as an ideal technique for obtaining early information. Quite often it is realized, although data can be acquired reasonably quickly, it takes far longer to process/analyze and create spatial information of relevance. Damage assessment can be easily carried out when pre and post event images are available. It can be assessed by using simple change detection techniques. But events like earthquake usually unpredictable in nature. So quite often pre event image data may not be available. Traditionally, image scales are used synonymously with image information. In a given image the highest level of data is present in the original resolution or scale. If we successively degrade the resolution then the coarser scale of one pixel value happens. At some scales some of the features just appear / disappear. These are called scale space events. Texture is spatial variation of gray tone. Finer texture implies high spatial variation. In the scale space methods, when one proceeds from finer scale to coarser scale, the fine structure (texture) disappear first followed by coarser texture. This idea has been used to separate standing buildings from the rubble. Area under the rubble is used as a measure of damage. The rubble is present in the form of fine texture, which can be separated by use of scale space representation. It is then followed by low level image processing techniques to form a continuous cluster of rubble, while eliminating the noisy regions. The assessed data by the proposed method was compared with visual interpreted damage area and found to be in correlation of 0.96. This method is very effective for estimation of damage area automatically and can be a very useful tool in applications of disaster management. This paper also addresses issues concerning optimal scale for the damage assessment. Remote sensing makes observation of any object from a distance and without coming into actual contact. Remote sensing can gather data much faster than ground based observation, can cover large area at one time to give a synoptic view. Remote sensing comprises Aerial Remote Sensing which is the process of recording information, such as photographs and images from sensor on aircrafts and Satellite Remote Sensing which consists of several satellite remote sensing system which can be used to integrate natural hazard assessments into development planning studies.These are: Landsat, SPOT Satellite, Satellite Radar System, Advanced Very High Resolution Radio. GIS and Remote Sensing can be used for preparing seismic hazards maps in order to assess the exact nature of risks. Landslide zonation maps comprise a map demarcating the stretches or area of varying degree of anticipated slope stability or instability. The map has an inbuilt element of forecasting and is hence of probabilistic nature. Depending upon the methodology adopted and the comprehensiveness of the input data used, a landslide hazard zonation map able to provide help concerning location,- extent of the slop area likely to be affected, and rate of mass movement of the slope mass. GIS can also be used in carrying out search and rescue operations in a more effective manner by identifying areas that are disasters prone and zoning them accordingly to risk magnitudes. In the present era of electronic communication, the internet provides a useful platform for disaster mitigation communications. Launching of a well defined web site is a very cost-effective means of making an intra-national and international presence felt. It provides a new and potentially revolutionary option for the rapid, automatic, and global dissemination of disaster information. A number of individuals and groups, including several national meteorological services, are experimenting with the Internet for real-time dissemination of weather observation, forecasts, satellite and other data. In the most critical phase of natural disasters electronic communication have provided the most effective and in some instances perhaps the only means of communication with the outside world. Opinion: Taking Action on Earthquakes in Iran Manuel Berberian, Ph.D. Earthquake Seismologist & Geoscientist Najarian Associates Inc. Manual Berberian is a project manager with 33 years of academic/field experience in Geophysics, Seismology and Applied Geology, and 8 years of experience in Environmental Science and Engineering (site investigations & remediation at ISRA, RCRA, UST, CERCLA, or Brown Field sites involving solid waste, soil, & groundwater). He has been working with scientists from University of Cambridge (UK), University of Oxford (UK), Oregon State University (Corvallis, OR, USA), Jet Propulsion Laboratory of Caltech/NASA (CA, USA), and UNESCO/IDNDR (Paris, France) on different international research projects in geology and geophysics. Dr. Berberian is one of the foremost experts on earthquake studies in Iran and has published numerous articles on the subject, some of which have been highlighted in the bibliography at the end of this document. Since the 1962 catastrophic earthquake in Iran, my long field/research experience shows that, the strategic actions formatted on papers, discussed in numerous conferences, and even creating building codes, have not ensured that earthquake hazard mitigation is effectively and practically incorporated in the Third World Countries' planning and retrofitting programs (if any). Hence, we are witnessing a cascading failure in this business, and should accept that we all were unable to break the disaster cycle in this field. The recent moderate magnitude earthquake damage at Bam (Iran) with widespread devastation (in a sparsely populated desert area), resembles a nuclear explosion with an unacceptable death toll, and is beyond imagination in the early years of the 21st century science and technology. Similar magnitude earthquake, which took place in central California on December 22, 2003, did not cause any dramatic damage or destruction with unimaginable death toll. The comparison clearly shows the failure of our efforts in this endeavor in the Third World Countries. We all should think critically and find new ways to break the disaster cycle in the Third World Countries, and make the governments liable for their failure. The moderate (Mw=6.6) Bam (SE Iran) earthquake of December 26, 2003 killed several thousands and demolished a city of 80,000 people located in a sparely populated area at the southwestern edge of the Lut Desert. Almost all public and commercial buildings were destroyed, lifelines were cut, and government quickly appealed to foreign governments (friends and foes) to supply help, equipment, food, medicine, and financial support. Sadly, the only two hospitals in Bam were collapsed and consequently, injured people were sent to far hospitals in provincial capitals, approximately hundreds of km away from the devastated city of Bam. This tragedy in unacceptable in the 21st century and one should search for its liability rather than foreign help. Major earthquakes in Iran have motivated Iranian earth scientists and engineers to map and study the seismogenic faults, estimate the seismic-fault hazard, and prepare building codes. It is more than three decades that we know the city of Bam is located next to the Bam seismogentic fault, and unfortunately the hospitals in this city were not reinforced. Essential buildings, in particular hospitals and health care facilities, are supposed to survive major earthquakes to provide service to injured people. Although the Iranian seismic code has addressed this basic concept, the policies have been enforced by the different governments and to take effective measures for preparedness for earthquakes. Foreign help cannot useful in the Third World countries; it is the government's responsibility to work seriously on earthquake hazard minimization. For more than forty years, editorials have been written, speeches delivered, reports and books published, funds granted, rules, regulations and laws promulgated on the subject of earthquakes and their risks, and foreign helps were funneled into the country after occurrences of large-magnitude earthquakes in Iran. Despite these, we still cannot minimize the death tolls and financial losses of earthquakes in Iran. During the June 20, 1990 Rudbar-Tarom earthquake (Mw=7.3) in northwest Iran, more than 40,000 people lost their lives, more than 500,000 became homeless, nearly 100,000 buildings were destroyed, three cities and 700 villages were razed to the ground. Such great disaster occurred not only because of a large magnitude earthquake but also because of poor construction and preparation in vulnerable areas. Reconstruction of the region was estimated to cost at least 2.8 billion dollars [economic losses inflicted by this earthquake were estimated $ 7.2 billion, with 7.2% in GNP loss]. The long-term effects of this catastrophic event included the disruption of the economies of at least three large provinces, and the human resettlement of at least three large cities and 700 villages; reconstruction to modern standards has taken decades to accomplish and absorbed a considerable part of the country's budget. Comparison of recent records on the impact of earthquake hazards of similar magnitude in countries of varying levels of development is astonishing. During the Ms=7.1 Loma Prieta, California earthquake of 1989.10.17, only 62 people were killed, which is a very low casualty figure for an earthquake of suchmagnitude. Compare this with the 12,200 deaths during the 1962.09.01 (Ms=7.2) Bo'in (Buyin) Zahra earthquake in Iran. The difference in casualty numbers between these two events is directly related to differences in disaster preparedness and disaster mitigation in the United States of America and Iran. Strict adherence to building codes during the past three decades in the San Francisco region undoubtedly saved many lives and kept thousands of buildings from collapsing in the Loma Prieta earthquake; no similar step was seriously undertaken in Iran. In California, building codes and geologic site investigations were increasingly accepted; Iran has no comparable public commitment, and many houses are built with unreinforced masonry. Despite the frequency of earthquakes in western United States, the number of deaths due to earthquakes has been very low in the past fifty years as compared with losses in Iranian earthquakes. In this century, earthquakes in North America alone have resulted in more than a 1,000 deaths, while at least 126,000 people have lost their lives during earthquakes in Iran. These losses cannot be justified in the light of existing scientific knowledge and expertise in disaster management. It is particularly painful that more than 60 million Iranians are trapped in a "vicious circle" of earthquake vulnerability. Unfortunately, little has been done to reduce the risk of earthquakes in urban areas of developing countries. Factors such as: (i) rapid urban growth, (ii) weak economy, (iii) lack of government funds to support earthquake hazard mitigation programs in cities, towns and villages, (iv) lack of seismic rehabilitation programs for upgrading all highly vulnerable public buildings and multiple family residential buildings, (v) inexpensive and poorly constructed private dwellings that often fail even in the absence of earthquakes, (vi) a tendency in government and general population to ignore the earthquake hazard due to more immediate and basic needs, (vii) lack of, or low awareness about the earthquake hazard, and (viii) lack of enforcement of existing building codes, could definitely affect earthquake risk management in developing countries. Iran has been host to a long series of large damaging earthquakes, many of them occurring within the 20th century. There have been roughly 126,000 deaths attributed to 14 earthquakes of magnitude ~7.0 (one 7.0 earthquake/7-yr), and 51 earthquakes of 6.0-6.9 (one/2-yr) that have occurred in Iran since 1900. During this period nine (9) cities were devastated (one city/10-yr). These earthquakes represent a mix of urban and rural events, with levels of documentation varying from one earthquake to another. Unfortunately, actual human and financial loss estimates are not available for the most Iranian earthquakes. Like many urban areas in developing countries, Iranian cities have earthquake risks that have increased significantly since the last major earthquake in each area. Until possibly the early 60's, the rate of investment in large urban and industrial developments in Iran was rather small and in some of its provinces, insignificant. Consequently there have essentially been no significant economic losses from the large number of damaging earthquakes that have occurred in this part of the world. Although during the first 60years the20th century earthquakes damage in economic terms has remained relatively small, with the massive investments of the last four decades in new urban and industrial centers, future earthquakes in Iran are likely to cause serious economic losses. The GeoHazards International workshop at Almaty, Kazakhstan (1996) concluded that half of the six million people in the capital cities of the five central Asian republics occupy buildings are extremely vulnerable to collapse during earthquakes with death tolls up to 135,000 people and at least 500,000 injuries. Similar study at Katmandu, Nepal (EERI Newsletter, 1998) showed that the next major earthquake could kill 40,000 people, seriously injure 100,000 and leave even more homeless. The vulnerability of the capital city of Tehran (with ~12,000,000 population), and other provincial cities such as Mashhad (~3,000,000), and Tabriz (~2,000,000), all located next to several mapped seismogentic faults with documented history of several large-magnitude earthquakes, is scary. The losses would have been much more severe if the earthquake had been centered in these mega cities. Major urban areas across the county are at high risk of being devastated by earthquakes and other natural hazards. Unfortunately, foreign helps (except the humanitarian relief/emergency portion), technical reports, conferences, and workshops have never been able to break the disaster cycle in the Third World Countries. A critical element in good governance is, possibly, missing. Retrofitting the critical structures, exploring disaster mitigation, planning, preparedness, response and recovery are responsibilities of the governments. References used in this document Bibliography Berberian, M., “Opinion: Earthquake Management in Iran”, Iran Analysis Quarterly, Vol. 1 No.2, January 2004 International Institute for Earthquake Engineering and Seismology website www.iiees.ac.ir/English/eng_index.html Jayant Sinha, Sri Sahendra Singh, Sri Indrajeet, Sri Tarun Kumar Evaluation of parameters controlling Earthquake Management System : An analytical approach using 3S' Technology GIS Development (2000) Tumialan, G., Nanni, A., “Strengthening: masonry walls. (walls).(unreinforced masonry structures)”, April 2003, Looksmart, April 2003 Neeraj Mishra', P.Suresh Kumar, R.Chandrakanthz, R.Ramachandran, Dr. R. Krishna , Automatic Assessment of Earthquake Damaged Area usini Scalespace classification techniques, Advanced Data Processing Research Institute, 2002 Bibliography on Earthquake Studies in Iran Journal of Seismology and Earthquake Engineering International Institute of Earthquake Engineering and Seismology Allen MB, Ghassemi MR, Shahrabi M, et al., Accommodation of late Cenozoic oblique shortening in the Alborz range, northern Iran, J STRUCT GEOL 25 (5): 659-672 2003 Amiri CG, Motamed R, Es-Haghi HR, Seismic hazard assessment of metropolitan Tehran, Iran, J EARTHQ ENG 7 (3): 347-372 JUL 2003 Asgary A, Willis KG, Household behaviour in response to earthquake risk: An assessment of alternative theories DISASTERS 21 (4): 354-365 DEC 1997 Asgary A, Willis KG, Estimating the benefits of construction measures to mitigate earthquake risks in Iran, ENVIRON PLANN B 24 (4): 613-624 JUL 1997 Bahrainy H, Urban, planning and design in a seismic-prone region (the case of Rasht in Northern Iran), J URBAN PLAN D-ASCE 124 (4): 148-181 DEC 1998 Berberian M, Jackson JA, Qorashi M, et al., The 1997 May 10 Zirkuh (Qa'enat) earthquake (M-w 7.2): faulting along the Sistan suture zone of eastern Iran, GEOPHYS J INT 136 (3): 671-694 MAR 1999 Berberian M, Yeats RS, Patterns of historical earthquake rupture in the Iranian plateau, B SEISMOL SOC AM 89 (1): 120-139 FEB 1999 Berberian M, Jackson JA, Fielding E, et al., The 1998 March 14 Fandoqa earthquake (M-w 6.6) in Kerman province, southeast Iran: re-rupture of the 1981 Sirch earthquake fault, triggering of slip on adjacent thrusts and the active tectonics of the Gowk fault zone, GEOPHYS J INT 146 (2): 371-398 AUG 2001 Berberian M, Yeats RS, Contribution of archaeological data to studies of earthquake history in the Iranian Plateau, J STRUCT GEOL 23 (2-3): 563-584 FEB-MAR 2001 De Martini PM, Hessami K, Pantosti D, et al., A geologic contribution to the evaluation of the seismic potential of the Kahrizak fault (Tehran, Iran), TECTONOPHYSICS 287 (1-4): 187-199 MAR 20 1998 Espandar R, Lotfi V, Razaqpur G, Influence of effective parameters of nonorthogonal smeared crack approach in seismic response of concrete arch dams, CAN J CIVIL ENG 30 (5): 890-901 OCT 2003 Espandar R, Lotfi V, Comparison of non-orthogonal smeared crack and plasticity models for dynamic analysis of concrete arch dams COMPUT STRUCT 81 (14): 1461-1474 JUN 2003 GAO LP, WALLACE TC, The 1990 Rudbar-Tarom Iranian Earthquake Sequence - Evidence For Slip Partitioning, J GEOPHYS RES-SOL EA 100 (B8): 15317-15332 AUG 10 1995 Ghaemian M, Ghobarah A, Nonlinear seismic response of concrete gravity dams with dam-reservoir interaction, ENG STRUCT 21 (4): 306-315 APR 1999 GILLARD D, WYSS M, Comparison Of Strain And Stress Tensor Orientation - Application To Iran And Southern California, J GEOPHYS RES-SOL EA 100 (B11): 22197-22213 NOV 10 1995 Jackson J, Priestley K, Allen M, et al., Active tectonics of the South Caspian Basin, GEOPHYS J INT 148 (2): 214-245 FEB 2002 Keshishian F, Political bias and nonpolitical news: A content analysis of analysis of an Armenian and Iranian earthquake in the New York Times and the Washington Post, CRIT STUD MASS COMM 14 (4): 332-343 DEC 1997 Koyi HA, Hessami K, Teixell A, Epicenter distribution and magnitude of earthquakes in fold-thrust belts: insights from sandbox models, GEOPHYS RES LETT 27 (2): 273-276 JAN 15 2000 Mirzaei N, Gao MT, Chen YT, Delineation of potential seismic sources for seismic zoning of Iran, J SEISMOL 3 (1): 17-30 JAN 1999 Mobarakeh AA, Rofooei FR, Ahmadi G, Simulation of earthquake records using time-varying Arma (2,1) model, PROBABILIST ENG MECH 17 (1): 15- 34 JAN 2002 Modarres M, Zarei B, Application of network theory and AHP in urban transportation to minimize earthquake damages, J OPER RES SOC 53 (12): 1308-1316 DEC 2002 Nadjafi I, Atef MR, Broumand B, et al., Suggested guidelines for treatment of acute renal failure in earthquake victims, RENAL FAILURE 19 (5): 655-664 1997 NATEGHIAN F, Seismic Strengthening Of 8-Story Rc Apartment Building Using Steel Braces, ENG STRUCT 17 (6): 455-461 JUL 1995 Sarkar I, Hamzehloo H, Khattri KN, Estimation of causative fault parameters of the Rudbar earthquake of June 20, 1990 from near field SHwave data, TECTONOPHYSICS 364 (1-2): 55-70 MAR 27 2003 Shahabpour J, The role of deep structures in the distribution of some major ore deposits in Iran, NE of the Zagros thrust zone, J GEODYN 28 (2-3): 237- 250 SEP-OCT 1999 Shomali ZH, Empirical Green's functions calculated from the inversion of earthquake radiation patterns, GEOPHYS J INT 144 (3): 647-655 MAR 2001 TADA T, SHIMAZAKI K, Source Directivity Revealed By Array Analysis Of Short-Period Teleseismic Body Waves - The 1990 Manjil, Iran, Earthquake J PHYS EARTH 42 (6): 455-460 1994 Tavakoli B, Ghafory-Ashtiany M, Seismic hazard assessment of Iran ANN GEOFIS 42 (6): 1013-1021 DEC 1999 Tehranizadeh M, Hamedi F, Influence of Iran's earthquake ground motion parameters on design spectra using deterministic and probabilistic approaches, J EARTHQUAKE ENG 7 (2): 275-295 APR 2003 Tehranizadeh M, Hamedi F, Influence of earthquake source parameters and damping on elastic response spectra for Iranian earthquakes, ENG STRUCT 24 (7): 933-943 JUL 2002 Tehranizadeh M, Passive energy dissipation device for typical steel frame building in Iran, ENG STRUCT 23 (6): 643-655 JUN 2001 Veiseh S, Yousefi AA, The use of polystyrene in lightweight brick production, IRAN POLYM J 12 (4): 323-329 JUL-AUG 2003 Viti M, Albarello D, Mantovani E, Classification of seismic strain estimates in the Mediterranean region from a 'bootstrap' approach GEOPHYS J INT 146 (2): 399-415 AUG 2001 Walker R, Jackson J, Baker C, Surface expression of thrust faulting in eastern Iran: source parameters and surface deformation of the 1978 Tabas and 1968 Ferdows earthquake sequences, GEOPHYS J INT 152 (3): 749-765 MAR 2003 Walker R, Jackson J, Offset and evolution of the Gowk fault, SE Iran: a major intra-continental strike-slip system, J STRUCT GEOL 24 (11): 1677- 1698 2002 Zamani A, Hashemi N, A comparison between seismicity, topographic relief, and gravity anomalies of the Iranian Plateau, TECTONOPHYSICS 327 (1-2): 25-36 NOV 30 2000 |